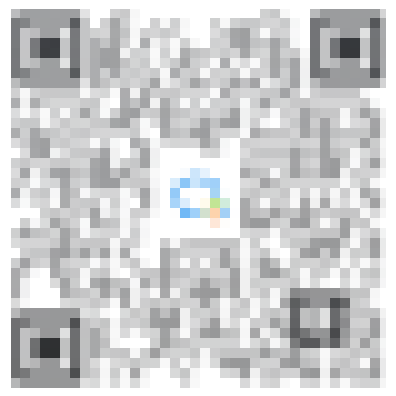
Scan the WeChat code to contact us
Scan the WeChat code to contact us
Feel free to send us a massage and we will reply to you as soon as possible.
Creating the future with heart and soul
As the core process for connecting and repairing cast steel parts, cast steel welding has a technical complexity due to the unique organizational characteristics and casting defects of cast steel materials. Cast steel parts often face technical challenges such as hydrogen-induced cracking, heat-affected zone embrittlement and residual stress concentration during welding due to high carbon equivalent, alloy element segregation and coarse grain structure. Based on the technical characteristics of five mainstream welding processes, this paper systematically discusses the process adaptability and quality optimization path of cast steel welding in combination with thermal cycle control, metallurgical behavior regulation and molten pool dynamics analysis. The study provides a theoretical basis and practical guidance for the selection of cast steel welding processes through parameter coupling optimization, operation mechanism analysis and engineering case verification.
1. Comparison of technical characteristics and adaptability analysis of welding processes
The selection of cast steel welding processes requires comprehensive consideration of material composition, structural geometric characteristics and service loads. With its process flexibility and simplicity of equipment, metallurgical arc welding (SMAW) has an advantage in casting repair and on-site operations. The core of the technology is to regulate the metallurgical reaction through the electrode coating. After baking, low-hydrogen electrodes (such as E5015) can effectively reduce the diffusible hydrogen content of the weld, and the crack resistance is improved by more than 40% compared with acid electrodes. However, the heat input fluctuation caused by the manual operation is easy to cause defects such as lack of fusion and slag inclusion, and the short arc operation specifications (arc length ≤ electrode diameter) and interlayer cleaning process must be strictly followed.
Gas-shielded welding (GMAW/MIG) achieves precise heat input through pulse current control. Its deposition efficiency (10-15kg/h) is significantly higher than that of electrode arc welding, and it is suitable for flat welding and batch welding of medium and thick plates. Inert gas protection (such as pure Ar) can isolate air oxidation. With the adjustment of pulse frequency (50-200Hz) and duty cycle (40-60%), the purity and density of the weld are significantly improved. However, its wind resistance is weak (auxiliary measures are required when the wind speed is >2m/s), which limits its application in outdoor operations.
Tungsten inert gas welding (GTAW/TIG) uses non-melting tungsten electrodes and argon protection to achieve low heat input welding. The width of the heat-affected zone can be controlled within 1mm, which is particularly suitable for high-alloy cast steel and thin plate welding. Its arc stability and weld formation quality are better than other processes, but the deposition rate is low (1-3kg/h) and the tungsten electrode loss increases long-term costs. It is more suitable for precision welding scenarios such as butt joints of cast steel thin plates or root welding.
Submerged arc welding (SAW) has become the preferred process for long straight welds of thick castings due to its high deposition efficiency (20-50kg/h). The flux composition has a significant effect on metallurgical reactions. The combination of sintered flux (such as SJ101) and H08MnA welding wire is suitable for low-carbon cast steel, while smelting flux (such as HJ431) improves crack resistance by enhancing the desulfurization effect. However, its equipment is bulky and limited to flat welding positions, and the groove processing accuracy and interlayer temperature (≤300℃) must be strictly controlled.
Flux-cored gas-shielded welding (FCAW) combines flux transition and gas shielding and has both self-shielding and gas-shielding modes. Self-shielding welding wire (such as E71T-1) is suitable for all-position welding and has strong wind resistance (withstands 5m/s wind speed), which is ideal for outdoor construction and complex structure repair. Its weld toughness is better than SMAW, but the spatter is more extensive, and the weld formation is rough. The process stability needs to be improved through parameter optimization (such as an extension length of 15-25mm).
2.Multi-dimensional analysis of process optimization strategy
The quality optimization of cast steel welding requires coordinated breakthroughs in three aspects: thermal cycle control, molten pool behavior regulation and deformation suppression. The core of thermal cycle control is to reduce the risk of diffusible hydrogen content and HAZ embrittlement. Through step preheating (150-300℃) combined with post-heating (200-350℃×2-4h), the crack rate can be reduced by more than 60%. Interlayer temperature control (≤400℃) combined with infrared temperature measurement real-time monitoring can effectively avoid grain coarsening. Experiments show that for every 50°C increase in preheating temperature, the crack resistance of the weld increases by 15%-20%.
Molten pool behavior control improves the fluidity of the molten pool by optimizing pulse parameters (frequency 50 200, duty cycle 40-60%). It controls the geometry of the molten pool with welding gun swing technology (such as a “zigzag” shape). Simulation results show that increasing the pulse frequency by 100Hz can reduce the molten pool width by 15%, increase the penetration depth by 8%, and significantly improve the density of the weld. For multi-layer welding, the single-layer thickness is recommended to be ≤4mm. The hammer method is used to eliminate residual stress, which can reduce the crack sensitivity by about 30%.
Deformation control needs to be combined with welding sequence optimization and fixture constraints. Symmetrical welding sequence and segmented skip welding can reduce deformation by 40%, and presetting the reverse deformation (taking 50-80% of the deformation) can further improve the accuracy. Finite element simulation shows that rigid fixtures can reduce angular deformation by more than 50%. For complex structures, real-time deformation monitoring and dynamic parameter adjustment strategies are recommended.
3.Engineering application and future development direction
Taking the crack repair of a large cast steel gear (material ZG34CrNiMo) as an example, pulse MIG welding (E71T-GS welding wire, φ1.2mm, current 160A, voltage 20V) was used, combined with 200℃ preheating and segmented jump welding method, the weld tensile strength reached 720MPa, yield strength 580MPa, -40℃ impact energy 85J, fully meeting the service requirements. This case verifies the synergistic effect of process parameters and operating specifications.
In the future, cast steel welding technology will develop in the direction of intelligence and precision. Digital welding technology (such as robot welding) combined with visual recognition and big data algorithms can realize online optimization of welding parameters and defect warnings. At the same time, the development of new welding materials (such as metal core welding wire) and composite shielding gas (such as Ar+He mixed gas) will further improve weld performance and process efficiency. Through the cooperation of industry, academia and research to promote technological innovation, cast steel welding is expected to achieve a broader range of application breakthroughs in the field of high-end equipment manufacturing.